Overview of Drone Battery Types and Chemistry
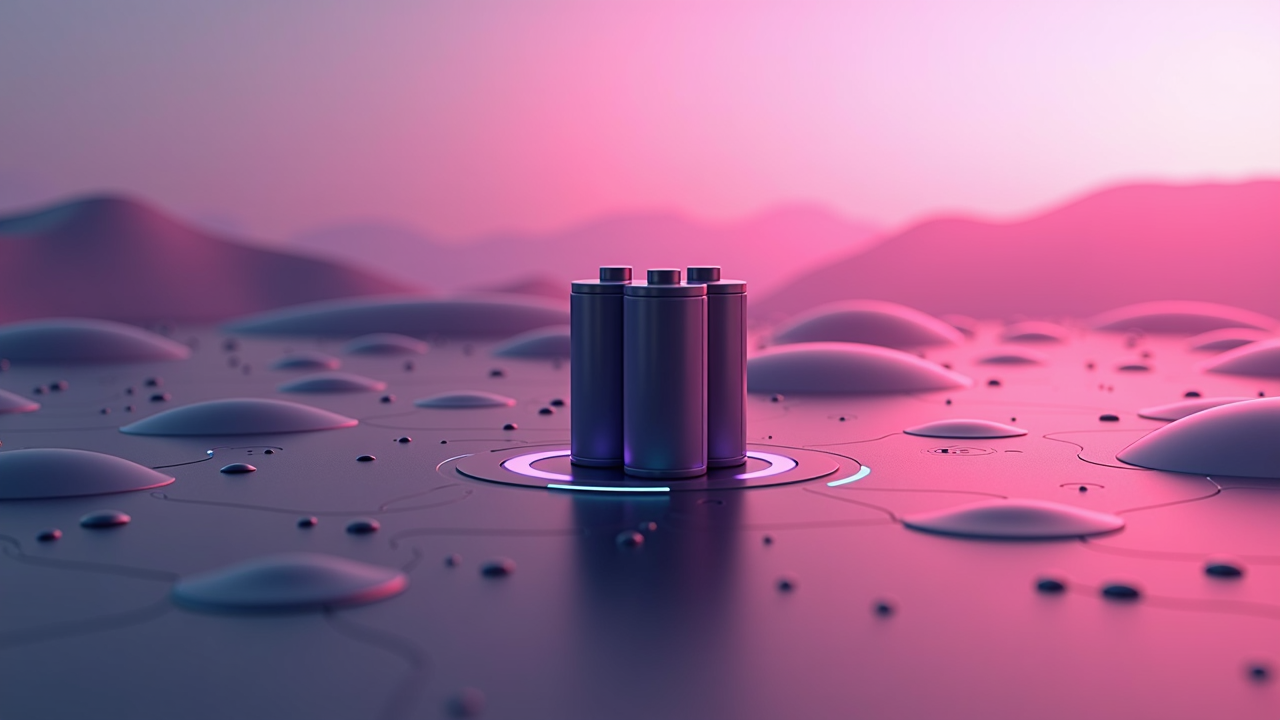
Batteries are the lifeblood of any drone, providing the power that keeps your aircraft in the air. Choosing the right battery is crucial for optimal performance, flight time, and safety. This comprehensive guide explores the different types of batteries used in FPV drones, their underlying chemistry, performance characteristics, and how to select the perfect power source for your specific needs.
Introduction to Drone Batteries
Drone batteries convert stored chemical energy into electrical energy that powers motors, flight controllers, cameras, and other onboard systems. While there are several battery technologies available, lithium-based batteries dominate the drone market due to their excellent energy density, discharge capabilities, and relatively light weight.
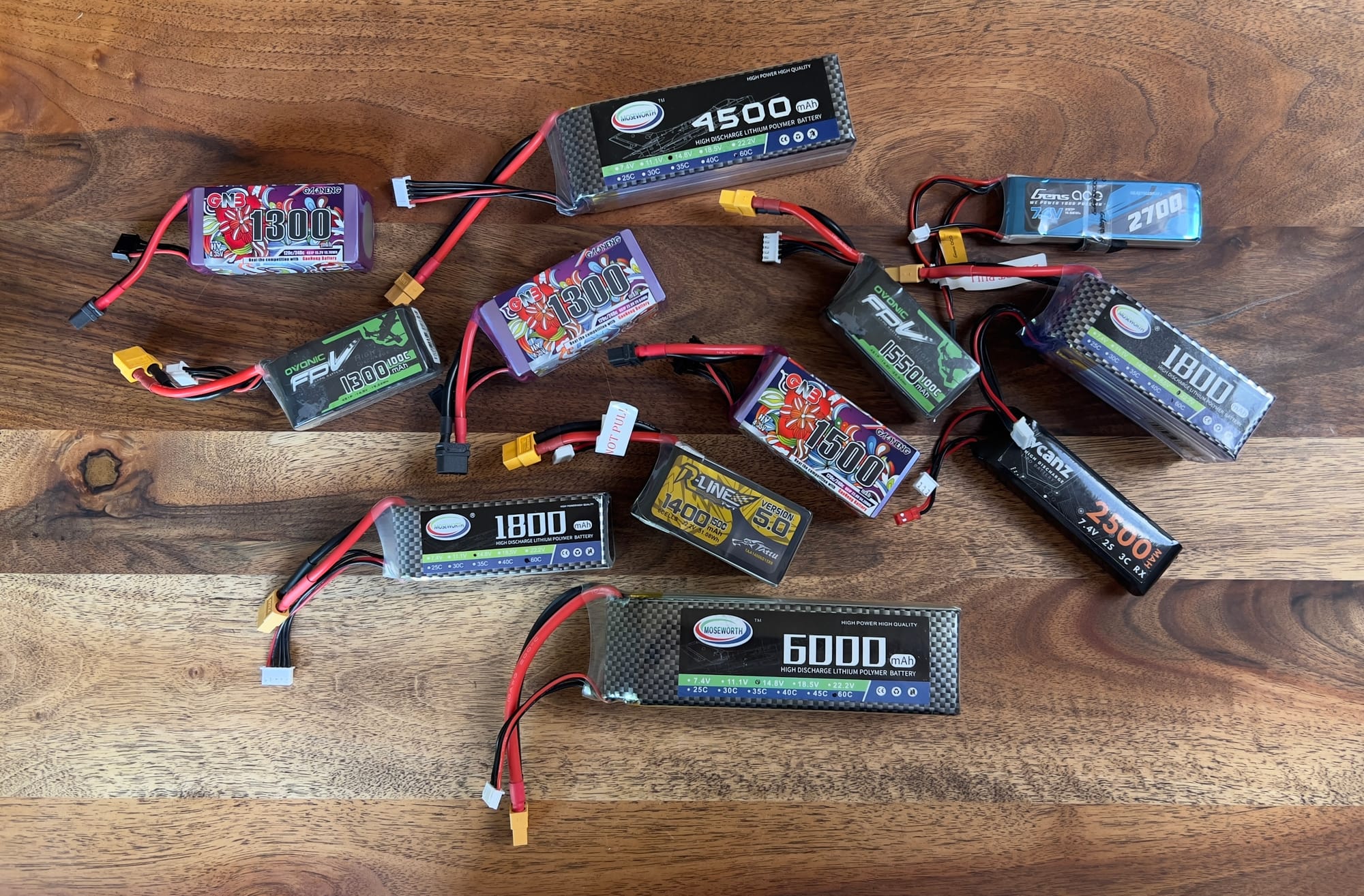
The Evolution of Drone Battery Technology
Battery technology has evolved significantly over the years, enabling longer flight times, higher performance, and more reliable operation:
- Early Days: The first consumer drones used Nickel-Cadmium (NiCd) or Nickel-Metal Hydride (NiMH) batteries, which were heavy and offered limited capacity.
- LiPo Revolution: The introduction of Lithium Polymer (LiPo) batteries revolutionized the hobby, offering higher energy density and discharge rates.
- Modern Advancements: Today's drones benefit from specialized LiPo variants like LiHV (High Voltage) and alternative lithium chemistries like Li-ion (Lithium-Ion) for specific applications.
Understanding Battery Chemistry
At their core, all lithium-based batteries work on similar principles but with important variations in their chemical composition that affect performance, safety, and longevity.
Basic Principles of Lithium Batteries
All lithium batteries consist of:
- Anode: Typically made of carbon (graphite) in most lithium batteries
- Cathode: Various lithium-containing compounds depending on the specific chemistry
- Electrolyte: The medium that allows lithium ions to move between the anode and cathode
- Separator: Prevents the anode and cathode from touching while allowing ions to pass through
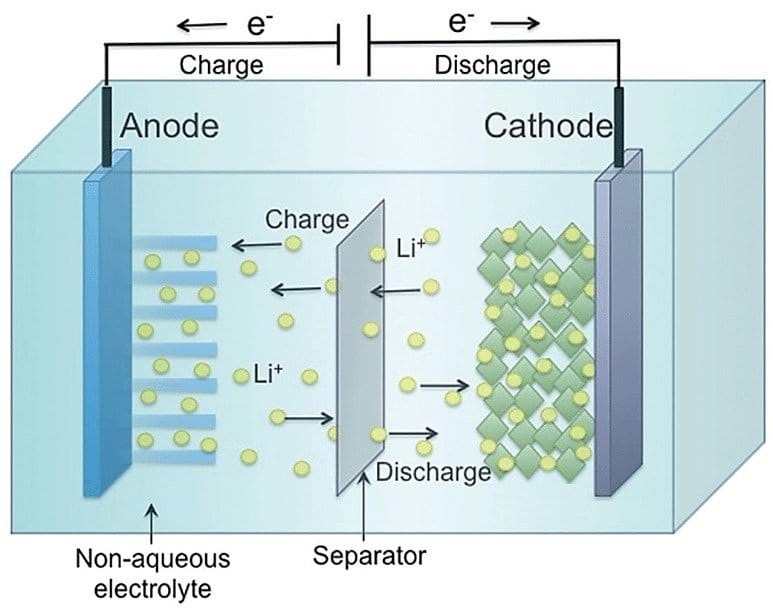
During discharge, lithium ions move from the anode to the cathode through the electrolyte, creating an electrical current in the external circuit. During charging, this process reverses, with lithium ions moving back to the anode.
LiPo (Lithium Polymer) Chemistry
LiPo batteries use a polymer electrolyte instead of a liquid electrolyte found in traditional lithium-ion batteries. The cathode is typically lithium cobalt oxide (LiCoO₂) or similar compounds.
Chemical Composition:
- Anode: Graphite (Carbon)
- Cathode: Lithium Cobalt Oxide (LiCoO₂) or similar lithium metal oxides
- Electrolyte: Polymer gel containing lithium salts
- Separator: Microporous polymer membrane
The chemical reaction during discharge can be simplified as:
LiC₆ + CoO₂ → C₆ + LiCoO₂
This chemistry provides:
- High energy density (130-200 Wh/kg)
- Excellent discharge rates (capable of 20C-100C or more)
- Relatively light weight
- Flexible form factor (can be manufactured in various shapes)
However, LiPo batteries are more volatile than other lithium chemistries and require careful handling to prevent thermal runaway (uncontrolled heating that can lead to fire).
LiHV (Lithium Polymer High Voltage) Chemistry
LiHV batteries are a variation of standard LiPo batteries with modified chemistry that allows for a higher maximum charge voltage.
Key Differences:
- Uses similar materials to standard LiPos but with additives that stabilize the electrolyte at higher voltages
- Can be charged to 4.35V per cell (vs. 4.20V for standard LiPos)
- Typically uses a slightly different cathode material that can handle higher voltages
The higher voltage provides approximately 8% more energy density, but at the cost of reduced cycle life due to increased stress on the battery components.
Li-ion (Lithium Ion) Chemistry
Li-ion batteries used in drones typically use a different cathode material than LiPos, often lithium nickel manganese cobalt oxide (NMC) or lithium iron phosphate (LiFePO₄).
Common Li-ion Chemistries:
- NMC (Lithium Nickel Manganese Cobalt Oxide)
- Chemical Formula: LiNiMnCoO₂
- Characteristics: Good balance of energy density, power, and longevity
- Common in: 18650 and 21700 cells used for long-range drones
- LFP (Lithium Iron Phosphate)
- Chemical Formula: LiFePO₄
- Characteristics: Lower energy density but extremely long cycle life and excellent safety
- Common in: Some specialized long-endurance applications
- NCA (Lithium Nickel Cobalt Aluminum Oxide)
- Chemical Formula: LiNiCoAlO₂
- Characteristics: Very high energy density but more temperature-sensitive
- Common in: High-end 18650 and 21700 cells
Li-ion batteries generally offer:
- Higher energy density than LiPo (150-260 Wh/kg)
- Lower discharge rates (typically 1C-10C)
- Longer cycle life (500-1000+ cycles vs. 200-300 for LiPo)
- Better safety profile with reduced risk of thermal runaway
LiPo Batteries: The Standard for FPV Drones
LiPo (Lithium Polymer) batteries are the most common type used in FPV drones due to their excellent balance of weight, power delivery, and energy density.
Voltage and Cell Count (S Rating)
LiPo batteries are made up of individual cells, each with a nominal voltage of 3.7V. The "S" rating indicates the number of cells connected in series:
- Nominal Voltage: 3.7V per cell (when approximately 50% charged)
- Fully Charged Voltage: 4.2V per cell
- Minimum Safe Voltage: 3.0V per cell (though landing at 3.5V per cell is recommended)
Common configurations include:
- 1S: 3.7V (4.2V fully charged) - Tiny Whoops and micro drones
- 2S: 7.4V (8.4V fully charged) - Micro drones and small 2"-3" quads
- 3S: 11.1V (12.6V fully charged) - Some micro quads and smaller 3"-4" quads
- 4S: 14.8V (16.8V fully charged) - Common in 5" freestyle and racing drones
- 6S: 22.2V (25.2V fully charged) - Increasingly standard for 5" freestyle and racing
- 8S: 29.6V (33.6V fully charged) - Larger drones for long-range or cinematography
- 12S: 44.4V (50.4V fully charged) - Very large drones for heavy lifting
State | Per Cell | 1S | 2S | 3S | 4S | 6S | Status/Action |
---|---|---|---|---|---|---|---|
Fully Charged | 4.20V | 4.20V | 8.40V | 12.60V | 16.80V | 25.20V | Ready to fly. Maximum energy available. |
90% Charged | 4.10V | 4.10V | 8.20V | 12.30V | 16.40V | 24.60V | Excellent power, slightly extended lifespan compared to full charge. |
80% Charged | 4.00V | 4.00V | 8.00V | 12.00V | 16.00V | 24.00V | Good power, better for battery longevity. |
Storage Voltage | 3.80-3.85V | 3.80-3.85V | 7.60-7.70V | 11.40-11.55V | 15.20-15.40V | 22.80-23.10V | Optimal voltage for long-term storage. Maximizes battery lifespan. |
Nominal Voltage | 3.70V | 3.70V | 7.40V | 11.10V | 14.80V | 22.20V | Approximately 50% charged. Reference voltage for specifications. |
40% Charged | 3.60V | 3.60V | 7.20V | 10.80V | 14.40V | 21.60V | Lower power available, consider landing soon. |
Warning Level | 3.50V | 3.50V | 7.00V | 10.50V | 14.00V | 21.00V | Recommended minimum during flight. Land soon to preserve battery health. |
Low Voltage | 3.30V | 3.30V | 6.60V | 9.90V | 13.20V | 19.80V | Very low power, potential for reduced battery lifespan if maintained. |
Minimum Safe | 3.00V | 3.00V | 6.00V | 9.00V | 12.00V | 18.00V | Absolute minimum. Risk of permanent damage below this level. |
Critical/Damage | <3.00V | <3.00V | <6.00V | <9.00V | <12.00V | <18.00V | Battery damage likely. Special recovery procedures required. |
LiHV Battery Voltages
State | Per Cell | 1S | 2S | 3S | 4S | 6S | Status/Action |
---|---|---|---|---|---|---|---|
Fully Charged | 4.35V | 4.35V | 8.70V | 13.05V | 17.40V | 26.10V | Ready to fly. Maximum energy available. |
90% Charged | 4.25V | 4.25V | 8.50V | 12.75V | 17.00V | 25.50V | Excellent power, slightly extended lifespan compared to full charge. |
80% Charged | 4.15V | 4.15V | 8.30V | 12.45V | 16.60V | 24.90V | Good power, better for battery longevity. |
Storage Voltage | 3.85-3.90V | 3.85-3.90V | 7.70-7.80V | 11.55-11.70V | 15.40-15.60V | 23.10-23.40V | Optimal voltage for long-term storage. Maximizes battery lifespan. |
Nominal Voltage | 3.80V | 3.80V | 7.60V | 11.40V | 15.20V | 22.80V | Approximately 50% charged. Reference voltage for specifications. |
40% Charged | 3.70V | 3.70V | 7.40V | 11.10V | 14.80V | 22.20V | Lower power available, consider landing soon. |
Warning Level | 3.50V | 3.50V | 7.00V | 10.50V | 14.00V | 21.00V | Recommended minimum during flight. Land soon to preserve battery health. |
Low Voltage | 3.30V | 3.30V | 6.60V | 9.90V | 13.20V | 19.80V | Very low power, potential for reduced battery lifespan if maintained. |
Minimum Safe | 3.00V | 3.00V | 6.00V | 9.00V | 12.00V | 18.00V | Absolute minimum. Risk of permanent damage below this level. |
Critical/Damage | <3.00V | <3.00V | <6.00V | <9.00V | <12.00V | <18.00V | Battery damage likely. Special recovery procedures required. |
Li-ion Battery Voltages
State | Per Cell | 1S | 2S | 3S | 4S | 6S | Status/Action |
---|---|---|---|---|---|---|---|
Fully Charged | 4.20V | 4.20V | 8.40V | 12.60V | 16.80V | 25.20V | Ready to fly. Maximum energy available. |
90% Charged | 4.10V | 4.10V | 8.20V | 12.30V | 16.40V | 24.60V | Excellent power, extended lifespan compared to full charge. |
80% Charged | 4.00V | 4.00V | 8.00V | 12.00V | 16.00V | 24.00V | Good power, optimal for battery longevity. |
Storage Voltage | 3.70-3.80V | 3.70-3.80V | 7.40-7.60V | 11.10-11.40V | 14.80-15.20V | 22.20-22.80V | Optimal voltage for long-term storage. Maximizes battery lifespan. |
Nominal Voltage | 3.60V | 3.60V | 7.20V | 10.80V | 14.40V | 21.60V | Approximately 50% charged. Reference voltage for specifications. |
40% Charged | 3.50V | 3.50V | 7.00V | 10.50V | 14.00V | 21.00V | Lower power available, consider landing soon. |
Warning Level | 3.40V | 3.40V | 6.80V | 10.20V | 13.60V | 20.40V | Recommended minimum during flight. Land soon to preserve battery health. |
Low Voltage | 3.20V | 3.20V | 6.40V | 9.60V | 12.80V | 19.20V | Very low power, potential for reduced battery lifespan if maintained. |
Minimum Safe | 2.80V | 2.80V | 5.60V | 8.40V | 11.20V | 16.80V | Absolute minimum. Risk of permanent damage below this level. |
Critical/Damage | <2.80V | <2.80V | <5.60V | <8.40V | <11.20V | <16.80V | Battery damage likely. Special recovery procedures required. |
Table 1. Standard lithium battery voltages
Capacity (mAh)
Capacity is measured in milliampere-hours (mAh), indicating how much current the battery can deliver for one hour:
- A 1000mAh battery can theoretically provide 1000mA (1A) for one hour
- Or 2000mA (2A) for 30 minutes, and so on
Higher capacity means longer flight times but also increased weight. Finding the optimal balance is crucial, as a heavier drone requires more power to fly.
C-Rating
The C-rating indicates the maximum safe continuous discharge rate of a battery:
Formula:
Maximum Continuous Discharge Current (Amps) = Capacity (Ah) × C-rating
Example:
A 1500mAh (1.5Ah) battery with a 100C rating can theoretically deliver:
1.5Ah × 100C = 150A
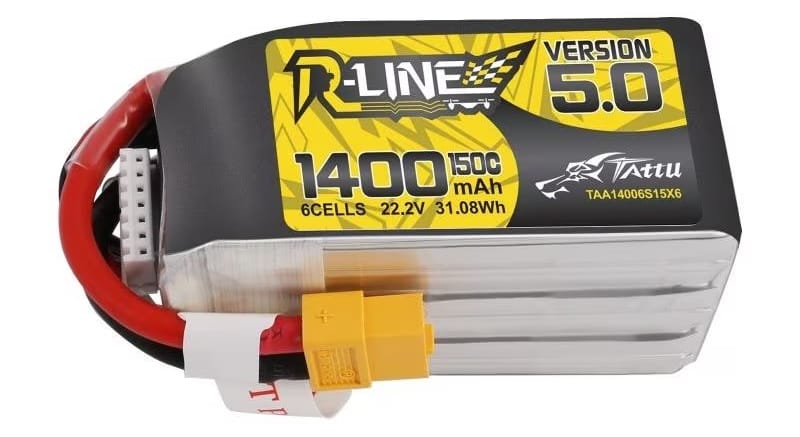
Important Note: Manufacturer C-ratings are often inflated. It's wise to be conservative in your calculations and choose batteries from reputable brands.
Internal Resistance (IR)
Internal resistance measures the opposition to current flow within the battery:
- Lower IR = more efficient power delivery, less voltage sag, and less heat generation
- Higher IR = reduced performance and shorter battery life
IR increases with age and usage, making it a good indicator of battery health:
- New, healthy battery: 1-5 milliohms per cell
- Aging battery: 10-15 milliohms per cell
- Worn-out battery: 20+ milliohms per cell
Connectors
Various connectors are used depending on the drone's size and power requirements:
- PH2.0: Very small connector for 1S tiny whoops
- BT2.0: Improved 1S connector with lower resistance than PH2.0
- GNB27: Another 1S connector similar to BT2.0
- A30: Newer 1S connector with even lower resistance
- JR / Futaba: RC receiver batteries, transmitter batteries, standard in RC industry for decades, used in radio systems and servo connection
- XT30: Small connector for 2S-4S batteries on smaller drones (up to 30A)
- XT60: Standard connector for 3S-6S batteries on larger drones (up to 60A)
- XT90: Heavy-duty connector for high-current applications (up to 90A)
For more detailed information on charging connectors, see:
Overview of Battery Charging Connectors
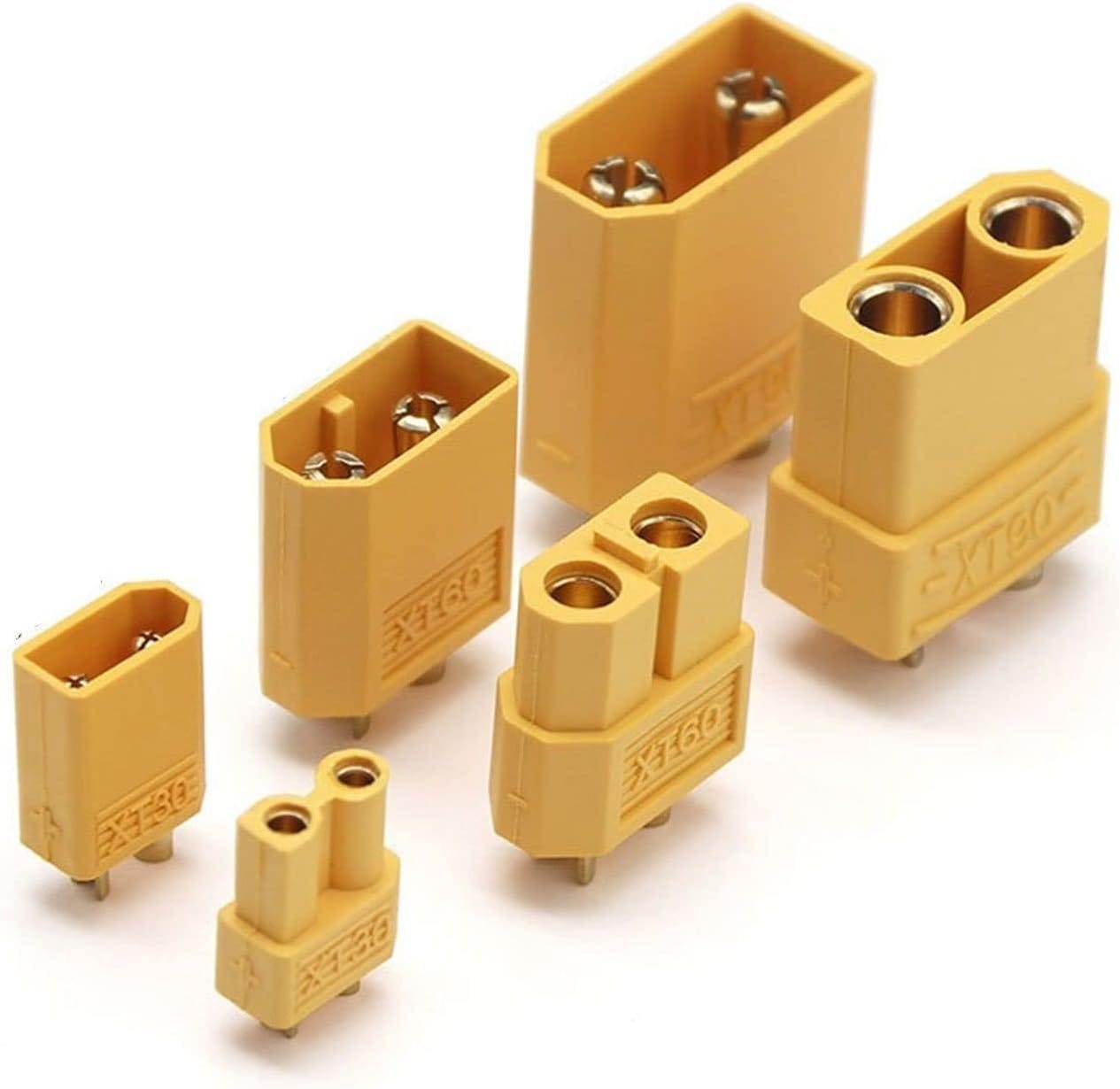
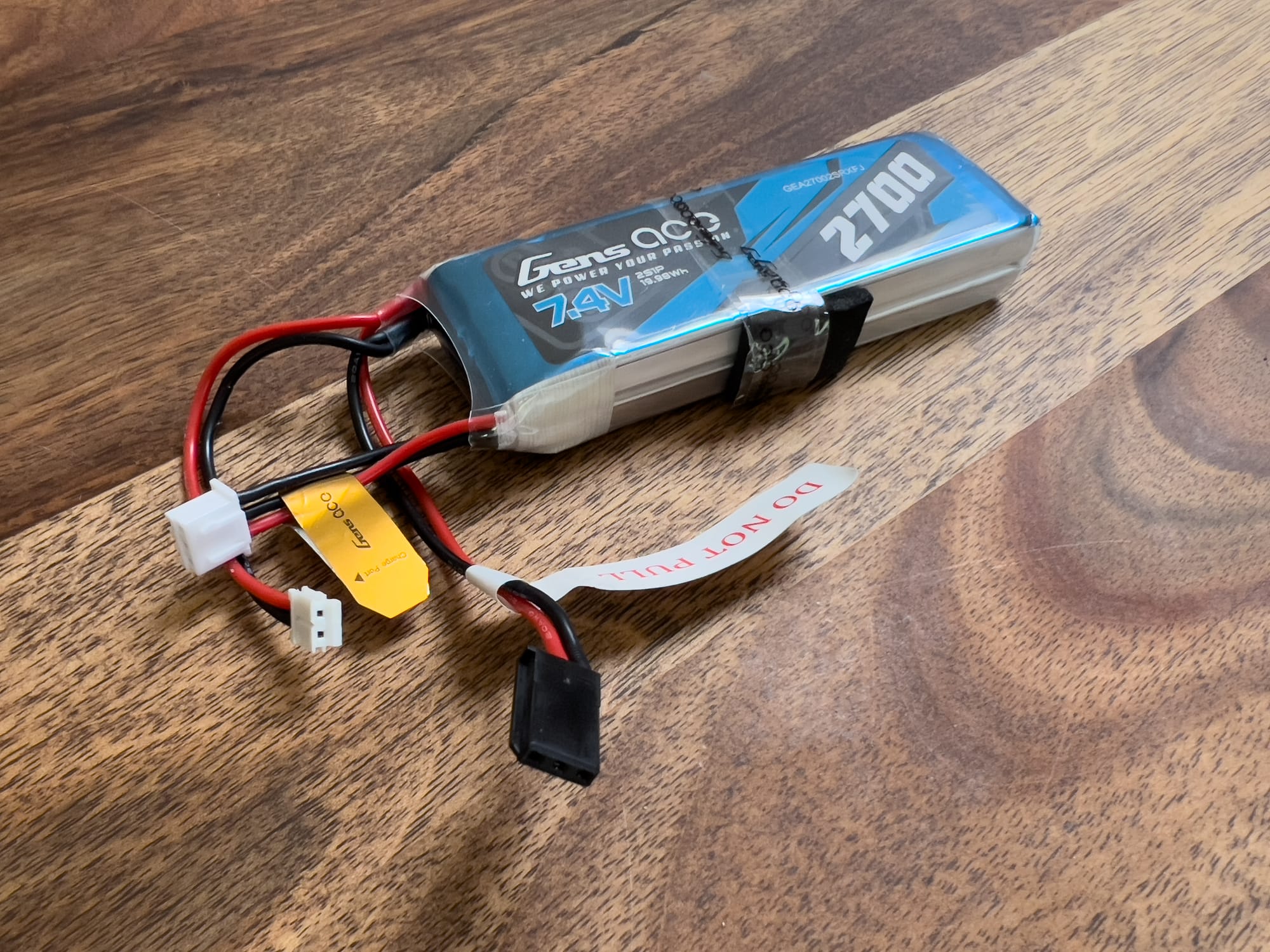
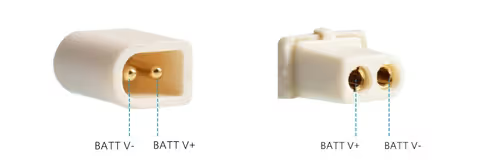
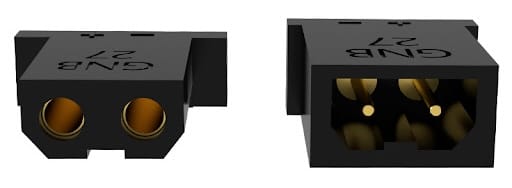
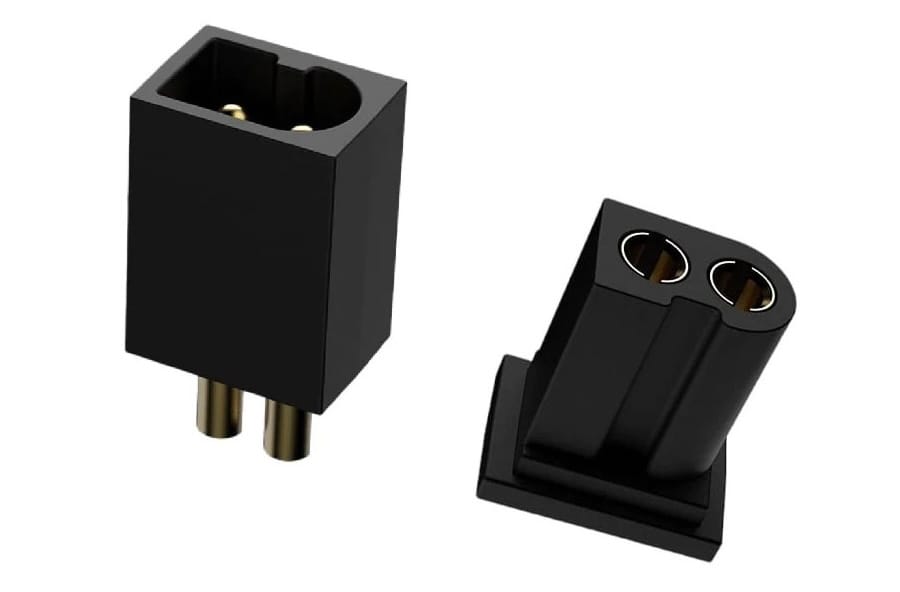
LiPo Battery Safety
LiPo batteries require careful handling due to their volatile chemistry:
Charging Safety:
- Use a dedicated balance charger and select the correct cell count
- Charge at 1C or less (1C = charging current equal to the battery's capacity in Ah)
- Never leave charging batteries unattended
- Use a fireproof container or LiPo bag
- Allow batteries to cool before charging
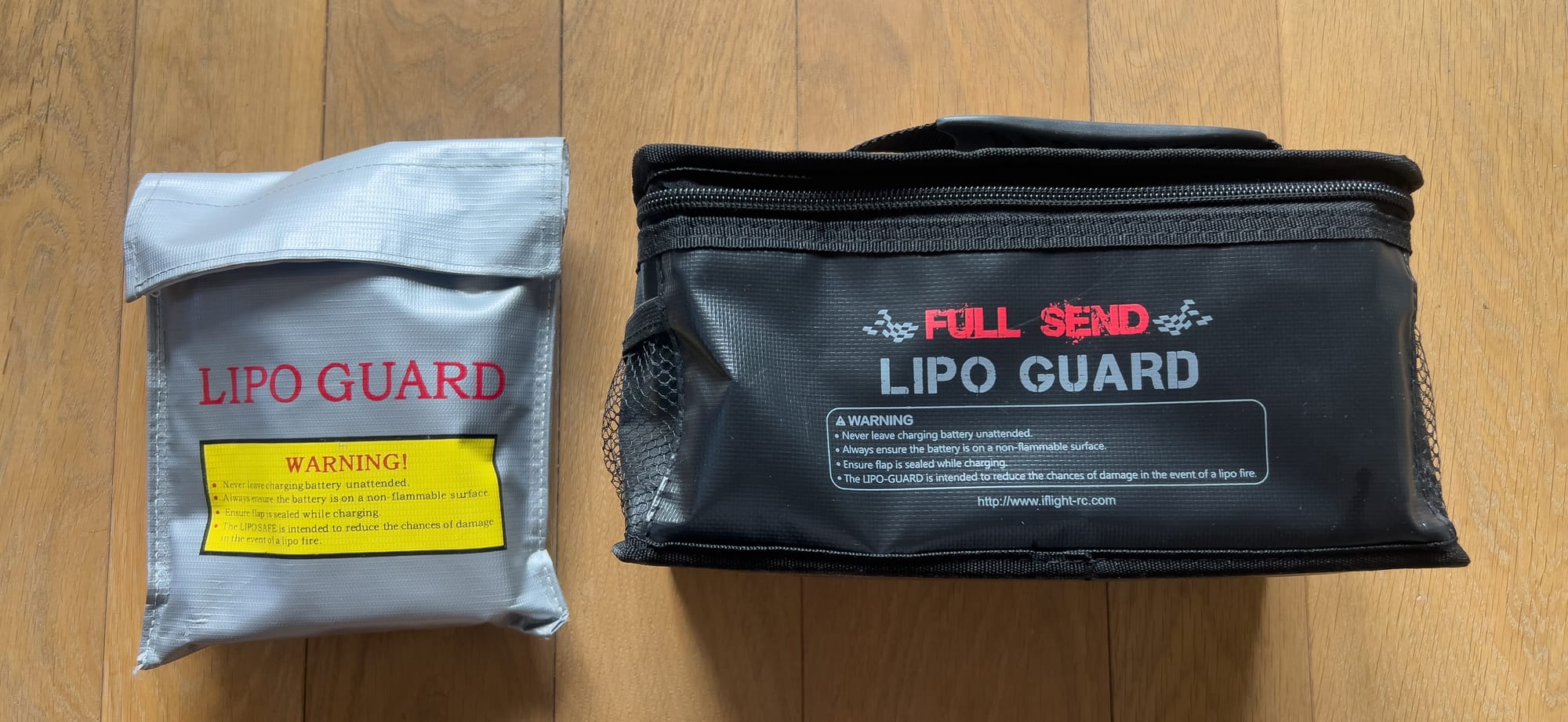
Storage:
- Store at 3.8-3.85V per cell (approximately 50% charged)
- Keep in a cool, dry place away from sunlight and flammable materials
- Use a LiPo-safe bag or container
Handling:
- Avoid physical damage (punctures, crushing, bending)
- Never use swollen or damaged batteries
- Secure batteries properly in your drone
- Prevent short circuits by covering connectors when not in use
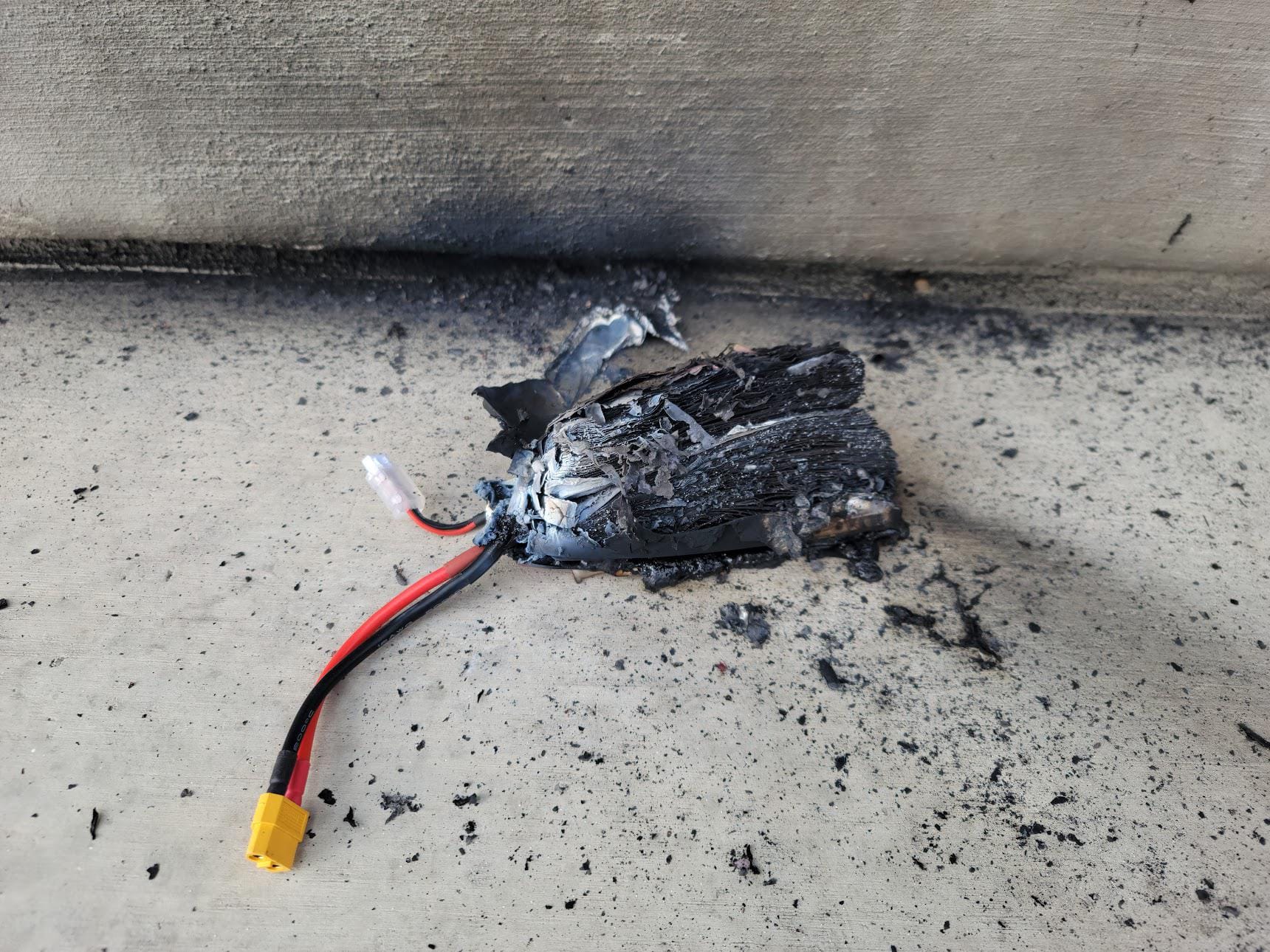
Disposal:
- Discharge to 3.0V per cell using a resistor or discharge function on your charger
- Submerge in salt water for 24+ hours to fully discharge
- Take to a battery recycling facility
For more detailed information on LiPo safety, see:
- Drone Battery Charging: A Guide to Safety and Efficiency
- Drone Battery Storage, Transport, and Discharge
- Parallel Battery Charging: Basic Principles and Advanced Techniques
LiHV Batteries: Higher Voltage Option
LiHV (Lithium Polymer High Voltage) batteries are a specialized variant of LiPo batteries that can be charged to a higher voltage.
Key Characteristics
- Maximum Cell Voltage: 4.35V (vs. 4.20V for standard LiPos)
- Energy Density: Approximately 8% higher than standard LiPos
- Performance: Slightly more power and potentially longer flight times, especially noticeable at the beginning of flights
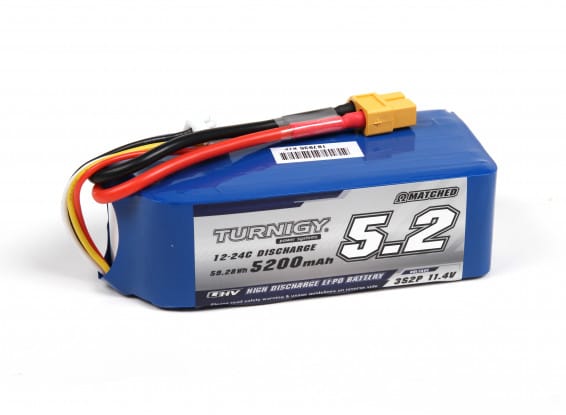
Advantages of LiHV
- Higher Voltage: Provides more power for the same weight
- Increased Energy Density: More flight time per gram of battery
- Performance Boost: Particularly beneficial for racing and tiny whoops where every bit of power matters
Disadvantages of LiHV
- Shorter Lifespan: The higher voltage stresses the cells, reducing cycle life by 20-30%
- Safety Concerns: Higher energy density means more potential energy release if something goes wrong
- Specialized Charging: Requires a charger that specifically supports LiHV mode
- Cost: Generally more expensive than standard LiPos
When to Choose LiHV
LiHV batteries make sense for:
- Racing drones where maximum performance is critical
- Tiny whoops and micro quads where the voltage boost is particularly noticeable
- Situations where the performance advantage outweighs the reduced lifespan
For general freestyle and recreational flying, standard LiPos often provide a better balance of performance, cost, and longevity.
Li-ion Batteries: The Long-Range Option
Li-ion (Lithium Ion) batteries offer higher energy density but lower discharge rates compared to LiPo batteries, making them ideal for long-range and endurance applications.
Key Characteristics
- Energy Density: 20-30% higher than LiPos (more capacity for the same weight)
- Discharge Rate: Typically 1C-10C (much lower than LiPos)
- Cycle Life: 500-1000+ cycles (2-3 times longer than LiPos)
- Voltage: Similar to LiPos (3.7V nominal, 4.2V fully charged per cell)
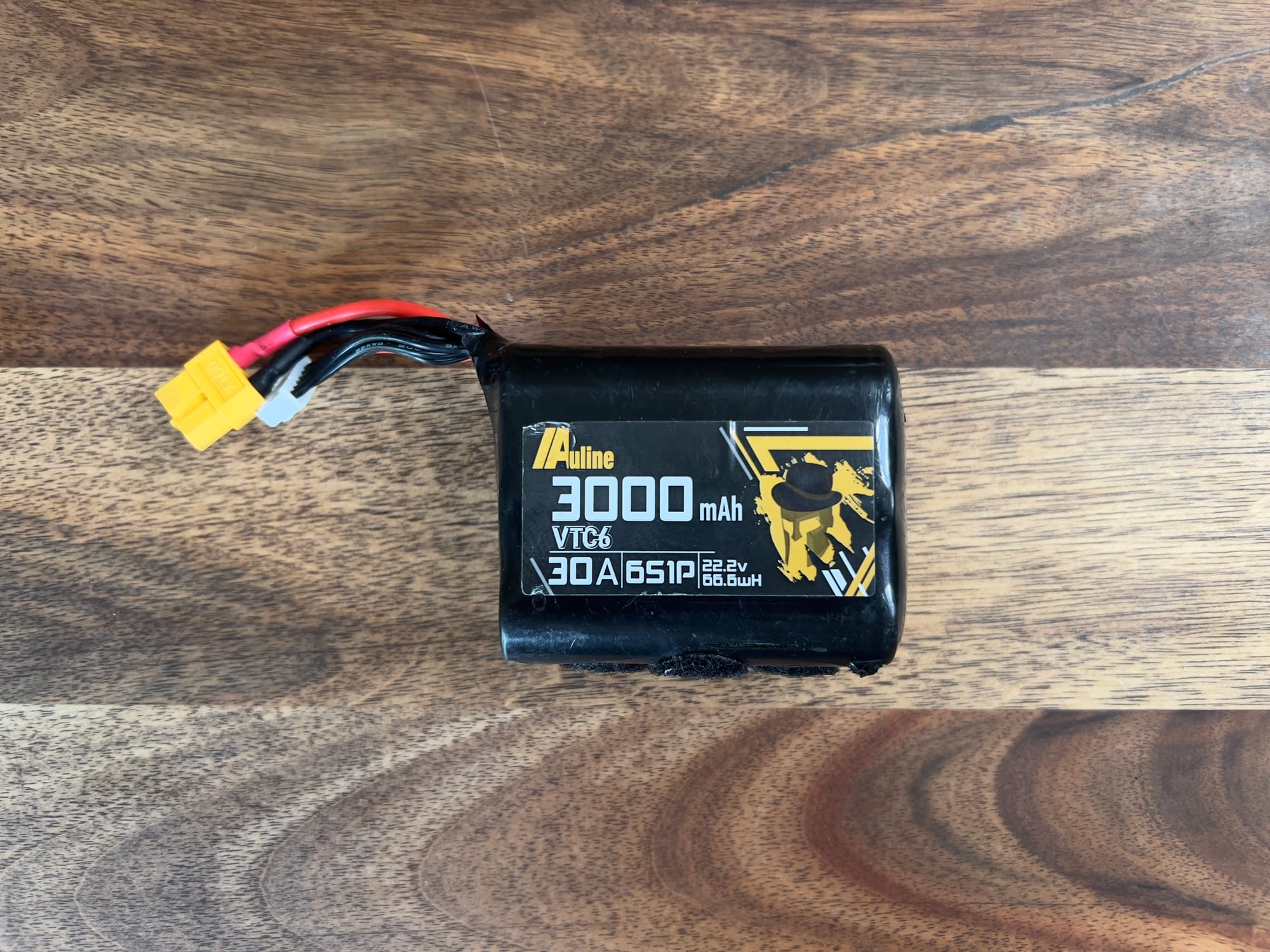
Common Cell Formats
- 18650: 18mm diameter, 65mm length, cylindrical format
- Capacity: 2000-3500mAh
- Discharge: 5-15A depending on the cell
- Common brands: Samsung, Sony, LG, Molicel
- 21700: 21mm diameter, 70mm length, cylindrical format
- Capacity: 3000-5000mAh
- Discharge: 10-30A depending on the cell
- Newer format with better performance than 18650
- 26650: 26mm diameter, 65mm length, cylindrical format
- Capacity: 4000-5500mAh
- Less common in drones due to weight
Advantages of Li-ion
- Longer Flight Times: The higher energy density translates directly to more time in the air
- Extended Lifespan: More charge cycles means better value over time
- Reduced Voltage Sag: Some Li-ion cells maintain voltage better under moderate loads
- Safety: Generally more stable and less prone to thermal runaway than LiPos
Disadvantages of Li-ion
- Limited Current Output: Cannot deliver the high currents needed for aggressive flying
- Weight Distribution: Cylindrical cells can be challenging to mount and balance
- Cost: Quality Li-ion packs are often more expensive initially (though potentially cheaper long-term)
- Complexity: Often requires custom battery packs and specialized knowledge
When to Choose Li-ion
Li-ion batteries are ideal for:
- Long-range missions where flight time is the priority
- Cruising-style flying rather than aggressive freestyle
- Larger drones where the weight distribution is less critical
- Applications where battery longevity and reliability are more important than maximum performance
Advanced Battery Concepts
For experienced pilots looking to optimise their setups, understanding these advanced concepts can help extract maximum performance from your batteries.
Parallel vs. Series Configurations
Batteries can be connected in different ways to achieve specific voltage and capacity characteristics:
- Series Connection (S): Connects the positive terminal of one battery to the negative terminal of another, increasing voltage while maintaining the same capacity.
- Example: Two 3S 1500mAh batteries in series = 6S 1500mAh
- Parallel Connection (P): Connects positive terminals together and negative terminals together, maintaining the same voltage but increasing capacity.
- Example: Two 3S 1500mAh batteries in parallel = 3S 3000mAh
- Series-Parallel Configurations: Combines both approaches for custom voltage and capacity.
- Example: Two 3S 1500mAh batteries in series, with two such pairs in parallel = 6S 3000mAh
For more detailed information on parallel charging, see:
Parallel Battery Charging: Basic Principles and Advanced Techniques
Battery Impedance and Performance
Impedance is a complex measure of a battery's resistance to current flow, including both resistive and reactive components:
- DC Resistance: The pure resistive component, measured as IR
- AC Impedance: Includes capacitive and inductive effects that vary with frequency
- Temperature Effects: Impedance increases at lower temperatures and decreases at higher temperatures
Understanding impedance helps explain why:
- Batteries perform better when warm (but not hot)
- Performance degrades in cold weather
- Older batteries experience more voltage sag
State of Charge (SoC) vs. State of Health (SoH)
Two important but distinct battery metrics:
- State of Charge (SoC): The current energy level of the battery (0-100%)
- Measured by voltage, but the relationship is non-linear
- More accurate when measured at rest than under load
- State of Health (SoH): The condition of the battery compared to its ideal specifications
- Affected by cycle count, storage conditions, and usage patterns
- Indicated by capacity retention and internal resistance
A new battery at 50% SoC will perform better than an old battery at 50% SoC due to differences in SoH.
Battery Memory Effect and Lithium Batteries
Unlike older NiCd batteries, lithium batteries do not suffer from true memory effect. However, they do experience:
- Voltage Depression: Temporary reduction in voltage after partial discharge/charge cycles
- Capacity Calibration Issues: Battery management systems may lose accuracy in estimating remaining capacity
Occasional full discharge cycles (to minimum safe voltage, not complete depletion) can help recalibrate battery management systems but aren't necessary for the health of the cells themselves.
For more detailed information on battery performance testing, see:
Battery Health Analysis
Practical Battery Selection Guide
Choosing the right battery involves balancing multiple factors based on your specific drone and flying style.
For Racing Drones
Priority: Maximum power delivery and quick response
- Battery Type: LiPo or LiHV
- Cell Count: 4S-6S depending on motor KV and ESC rating
- Capacity: Lower capacity (1000-1300mAh for 5" quads) to minimize weight
- C-Rating: 75C+ for immediate power delivery
- Considerations: Accept shorter flight times (2-3 minutes) in exchange for maximum performance
For Freestyle Drones
Priority: Balance of power, flight time, and handling
- Battery Type: LiPo
- Cell Count: 4S-6S depending on motor KV and flying style
- Capacity: Medium capacity (1300-1800mAh for 5" quads)
- C-Rating: 50C+ for good power delivery with reasonable weight
- Considerations: Look for batteries with consistent performance throughout the discharge cycle
For Long Range Drones
Priority: Maximum flight time and efficiency
- Battery Type: Li-ion or high-capacity LiPo
- Cell Count: 4S-6S depending on motor efficiency
- Capacity: High capacity (18650/21700 Li-ion pack or 2000mAh+ LiPo for 5" quads)
- C-Rating: 15C+ for Li-ion, 25C+ for LiPo
- Considerations: Optimize for cruise efficiency rather than maximum power
For Cinewhoops and Camera Drones
Priority: Smooth power delivery and reliability
- Battery Type: LiPo
- Cell Count: 3S-4S for smaller cinewhoops, 6S for larger camera rigs
- Capacity: Sized appropriately for the weight and desired flight time
- C-Rating: 40C+ for consistent performance
- Considerations: Voltage stability is crucial for clean video and reliable gimbal operation
For Tiny Whoops
Priority: Lightweight with adequate power
- Battery Type: 1S LiPo or LiHV
- Capacity: 300-450mAh depending on motor size
- C-Rating: 30C+ for good performance
- Connector: PH2.0, BT2.0, GNB27, or A30 depending on your setup
- Considerations: Multiple batteries are essential due to short flight times
Pro Tips for Battery Management
These advanced techniques can help you maximize performance and extend the life of your drone batteries.
Optimizing Battery Performance
- Pre-flight Warming: In cold weather, keep batteries warm (around 25°C/77°F) before flying for better performance
- Rest Period: Allow 5-10 minutes of rest after charging before flying for more stable voltage
- Post-flight Cooling: Let batteries cool for 15-20 minutes after flying before recharging
- Balanced Cells: Use a good balance charger and regularly check cell voltage differences (should be within 0.01-0.02V)
Extending Battery Lifespan
- Avoid Extreme Discharges: Land when you reach 3.5-3.6V per cell under load
- Proper Storage: Store at 3.8-3.85V per cell in a cool place (15-20°C/59-68°F)
- Cycle Management: Avoid unnecessary charge cycles; it's fine to recharge a partially used battery
- Temperature Control: Avoid exposing batteries to temperatures above 40°C/104°F or below 0°C/32°F
Battery Pack Building and Customization
For advanced users who want to build custom battery packs:
- Cell Matching: Select cells with similar internal resistance and capacity
- Parallel Groups First: When building series-parallel packs, create parallel groups first, then connect them in series
- Robust Connections: Use appropriate gauge wire and secure soldering techniques
- Protection Circuits: Consider adding a battery management system (BMS) for Li-ion packs
- Structural Support: Provide adequate physical protection and strain relief for connections
Battery Logging and Analysis
Tracking battery performance over time can provide valuable insights:
- Flight Logs: Record flight times, discharge rates, and minimum voltages
- IR Tracking: Measure internal resistance periodically to identify degradation
- Performance Charting: Plot capacity vs. cycle count to visualize battery aging
- Temperature Monitoring: Use an infrared thermometer to check for hotspots during charging/discharging
Troubleshooting Common Battery Issues
Even with proper care, battery problems can arise. Here's how to diagnose and address common issues.
Puffed/Swollen Batteries
Causes:
- Overcharging
- Over-discharging
- Physical damage
- Age-related degradation
- Excessive heat during use or charging
Solutions:
- Retire the battery immediately - swollen batteries are unsafe to use
- Discharge to storage voltage (3.8V per cell) if possible
- Dispose of properly following safety guidelines
Voltage Sag During Flight
Causes:
- Battery aging (increased internal resistance)
- Undersized battery for the current draw
- Cold temperature operation
- Poor connections or inadequate wiring
Solutions:
- Check and clean all connections
- Use thicker wires or better connectors if appropriate
- Consider a battery with higher C-rating or lower internal resistance
- Keep batteries warm before flying in cold weather
Imbalanced Cells
Causes:
- Cell damage
- Manufacturing defects
- Uneven discharge patterns
- Aging battery
Solutions:
- Balance charge at a slow rate (0.5C)
- If imbalance persists (>0.05V difference between cells), retire the battery
- For minor imbalances, storage at proper voltage for several days may help
Reduced Flight Time
Causes:
- Battery capacity degradation
- Increased power requirements (added weight, damaged props)
- Cold weather operation
- Aggressive flying style
Solutions:
- Check actual capacity during discharge with a battery analyzer
- Inspect drone for mechanical issues increasing power consumption
- Consider replacing the battery if capacity has dropped below 80% of original
Charging Problems
Causes:
- Charger issues
- Balance lead problems
- Damaged cells
- Extreme temperature
Solutions:
- Verify charger functionality with a known good battery
- Check balance leads and main power leads for damage
- Ensure charging at room temperature (15-25°C/59-77°F)
- Try a different charger if problems persist
FAQ: Common Questions About Drone Batteries
What does "S" mean in a LiPo battery?
The "S" rating indicates the number of cells connected in series. Each cell has a nominal voltage of 3.7V, so a 4S battery has a nominal voltage of 14.8V (4 × 3.7V).
What is the difference between LiPo and LiHV?
LiHV batteries can be charged to a higher voltage per cell (4.35V) compared to standard LiPo batteries (4.20V). This results in approximately 8% more energy density but typically reduces the battery's lifespan.
Can I use a 6S battery on a drone designed for 4S?
Generally, no. Using a higher voltage battery than your components are rated for can damage ESCs, motors, and electronics. However, it might be possible with throttle limiting in the flight controller and appropriate motor KV selection.
How do I know when to land my drone?
It's recommended to land when the battery voltage reaches around 3.5V per cell under load. Most flight controllers can be configured to provide voltage warnings through OSD displays or audible alerts.
How should I store my LiPo batteries?
Store LiPo batteries at a storage voltage of around 3.8V to 3.85V per cell, in a cool, dry place, and preferably in a fireproof container or LiPo bag.
What is "C-rating" and how does it affect battery performance?
The C-rating indicates the battery's maximum safe continuous discharge rate. A higher C-rating means the battery can deliver more current, which is important for high-performance drones requiring rapid power delivery.
What is "IR" and why is it important?
IR (Internal Resistance) measures the resistance to current flow within the battery. Lower IR means less power is lost as heat and the battery can deliver power more efficiently, resulting in better performance and less voltage sag.
Are Li-ion batteries better than LiPo for all applications?
No. While Li-ion batteries offer higher energy density and longer lifespan, they cannot deliver the high current needed for aggressive flying. They're better for long-range and endurance applications, while LiPo batteries remain superior for racing and freestyle.
How many charge cycles can I expect from my batteries?
- LiPo batteries: Typically 200-300 cycles with proper care
- LiHV batteries: Usually 150-250 cycles
- Li-ion batteries: Generally 500-1000+ cycles
Actual cycle life depends on how the battery is used and maintained.
Can I mix different battery brands or specifications on my drone?
It's not recommended to mix batteries with different specifications (capacity, C-rating, age) as this can lead to imbalanced performance and potential safety issues. Stick to identical batteries for optimal results.
Conclusion
Battery technology is a fascinating and rapidly evolving field that directly impacts the performance, safety, and enjoyment of your drone flying experience. By understanding the chemistry, specifications, and practical considerations of different battery types, you can make informed decisions that optimize your setup for your specific needs.
Whether you're racing at maximum speed, capturing cinematic footage, or exploring long-range capabilities, selecting the right battery is as crucial as any other component on your drone. Remember that proper care and handling not only extend the life of your batteries but also ensure your safety while enjoying this exciting hobby.
As battery technology continues to advance, we can look forward to even better performance, longer flight times, and enhanced safety features. Stay informed about new developments, and don't hesitate to experiment with different battery options to find the perfect match for your flying style.
Comments ()